Figure 1: Smouldering wildfire of a carbon-rich soil system in Las Tablas de Daimiel (Photo by Dr G Rein, 2009).
Researchers at Imperial College Hazelab, including members of Leverhulme Wildfires, have recently published a new paper developing a multi-step reaction model to study the potential of peatland fires starting from self-heating ignition, in the International Journal of Wildland Fire.
As an organic porous material, peat is prone to self-heating ignition, the type of spontaneous initiation of fire that can take place at ambient temperature and without any external event. These type of fires are rare, but can lead to catastrophic consequences: the ignition and subsequent smouldering combustion of peat can lead to large-scale peatland fires, causing environmental and climate damage (see Figure 1 above).
Peat is prone to self-heating ignition which, although rare, can lead to large-scale peatland fires, causing environmental and climate damage
Studying of this phenomena at an experimental level is very difficult, because large ecosystems cannot be tested in the lab, and so experimental studies of self-heating ignition of peat have focused on sizes that are testable in a lab, up to a few kilograms of peat. Therefore modelling tools are needed to have a better understanding of how this phenomena upscales to the field. Many methods used to upscale experimental results to natural peatland sizes, such as the Frank-Kamenetskii method, require assumptions such as the fact that the self-heating ignition is not limited by oxygen access, and the reaction rate is independent of oxygen. However, natural peatland layers are normally metres deep and they have a large tortuosity that slows down the oxygen diffusion from the ambient surface to deep down in peat layers. Therefore, oxygen access at the bottom of the large peat layer is limited.
Further difficulties in modelling the phenomena lie in the fact that in the natural environment, peat not only undergoes oxidation reactions, but also undergoes biological reactions as well as effects from the amount of moisture present in the peat. In fact, at low temperatures (usually < 100 °C), the respiration and growth of micro-organisms can generate a significant amount of heat and this overall exothermic process in peat is referred to as biological reaction. At high temperatures (when micro-organisms die), the chemical oxidation of the biomass accelerates drastically (due to the Arrhenius’s effect) and becomes the dominant exothermic process.
Therefore, when studying peat reactions, we have three different types of reactions we need to be concerned about, and their effects on the propensity of the peat to ignite and smoulder:
1) Biological activity at low temperature, which causes exothermic reactions that dominate the reactivity of peat below 60°C, caused by micro-organisms.
2) Moisture content of the peat, namely the amount of water present in the peat, that leads to drying reactions (endothermic reactions) for the water to evaporate at and above 100°C.
3) The chemical oxidation of the carbon in the peat, which is exothermic and dominates the reactivity of the peat at higher temperatures.
This is the first time that a multi-step scheme is used to simulate the self-heating ignition of smouldering peat fires, and may help in the prevention and mitigation of these wildfires
In the work led by Dr Han Yuan at Imperial Hazelab to give a better prediction of the ignition temperature and transient characteristics during peat self-heating ignition, a computational model that can simulate the complex physical and chemical processes (i.e. mass transfer, heat transfer and multi-step reactions) was made.
In the recently published paper, we build such a model based on the open-source code Gpyro and incorporate a 3-step reaction scheme (including both biological reaction, chemical oxidation, and drying) to study the multi-step effect. We validate the model against microscale and mesoscale experiments (Figure 2) that were carried out experimentally in 2017 using oven basket experiments as shown in Figure 3, and, based on the validated model, we simulate the self-heating ignition of field-size peat layers for the first time.
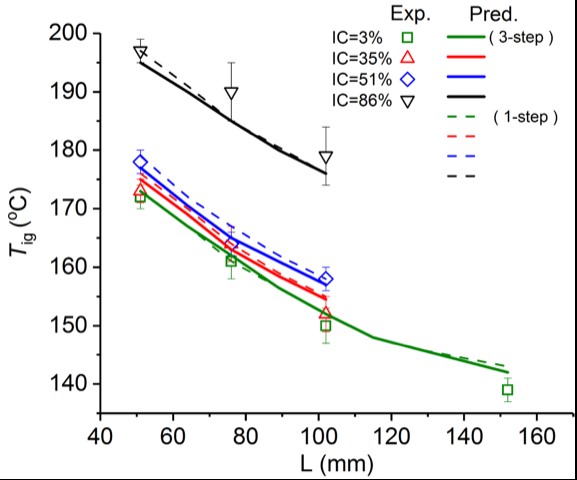
Figure 2. Comparison of experimental data (Restuccia et al. 2017) and simulations for samples with different sizes: symbols represent experimental data, lines represent simulation (the solid lines for 3-step scheme and the dotted lines for 1-step scheme ); Different colours refer to different mass fractions of sand in the sample. IC= inorganic content.
The work demonstrates that the model can predict correctly the transient temperature change, the time to ignition, and critical ignition temperature for peatlands.
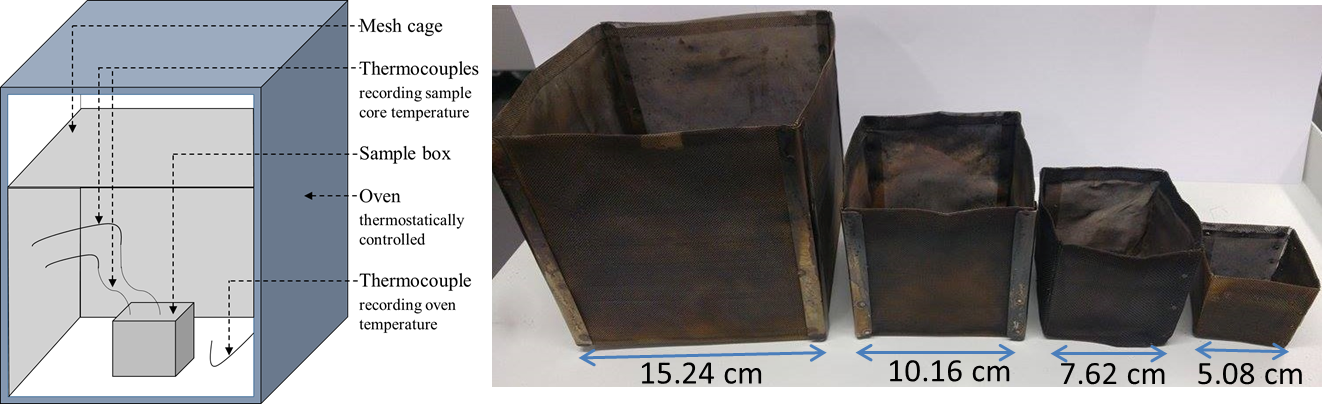
Figure 3. Experimental setup for meso-scale peat self-heating experiments (Restuccia et al 2017).
We show that the rapid depletion of oxygen in the sample is caused by chemical oxidation when approaching ignition conditions, because most of microorganisms die in the pre-drying process and the biological activities stop at high temperatures. In these conditions, the chemical oxidation accelerates rapidly (Figure 4), and the circular reaction zone moves from the centre to the periphery. This is because the oxygen is depleted at the centre area, so the reaction zone spreads to the free surface seeking for oxygen supply.
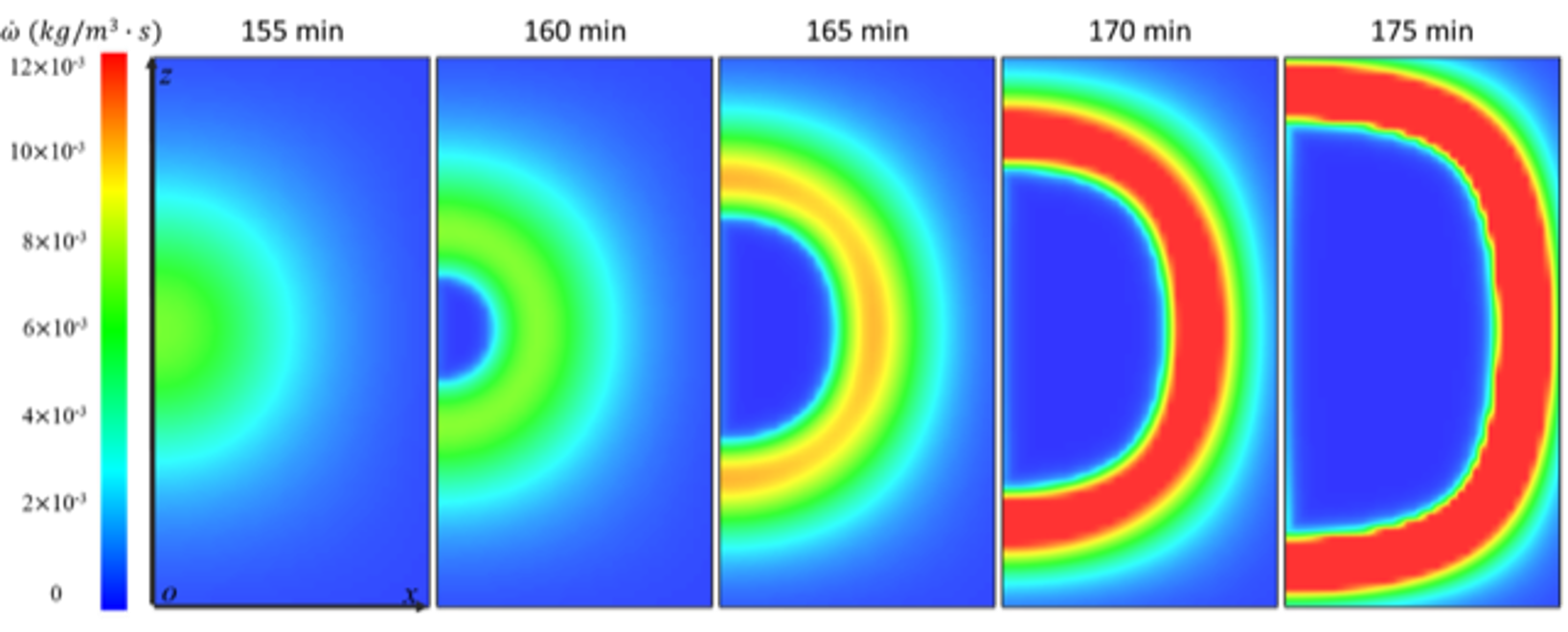
Figure 4. Transient reaction rate profiles of chemical oxidation at temperatures approaching ignition conditions.
Based on the validated model, we predict that a 1.5 m deep peat layer can be self-ignited under an average ambient temperature of 40 oC for inorganic contents ranging 3% to 45% at dry conditions and 3% to 20% in wet conditions (Figure 5).
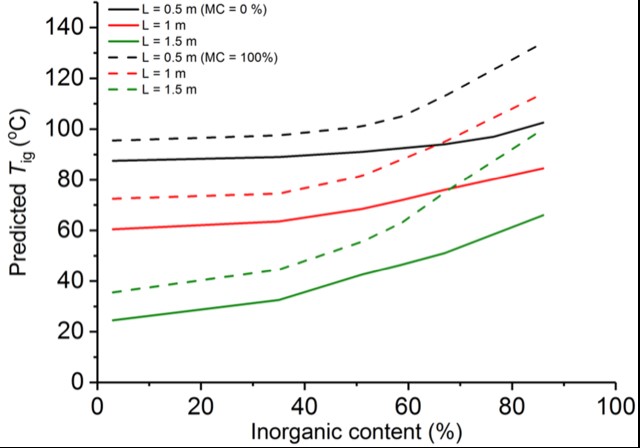
Figure 5. Comparison of predicted Tig (ignition temperature) for dry peat layers (Moisture Content, MC = 0%) and wet peat layers (MC = 100%) by 3-step reaction scheme; Inorganic content ranging from 3% to 86%
A further analysis on the multi-step effect reveals that the heat generated by biological reaction can accumulate in field-scale peat layer and then trigger the oxidative reaction, which eventually leads to the self-heating ignition of peat layers. This work is the first time that a multi-step scheme is used to simulate the self-heating ignition of smouldering peat fires, and aims to help in the prevention and mitigation of these wildfires.
Written by the study’s co-author Dr Francesco Restuccia, Lecturer in Engineering, King’s College London.